Oxidative stress is the oxidative modification of biological components in the body, such as proteins, nucleic acids and lipids. Since these biological substances are an important part of the body, their oxidation causes various organ dysfunctions. The “Free Radical Theory of Aging” points out that the accumulation of a series of such damages is the cause of the decline in age-related biological functions (aging). However, not all free radicals are harmful substances. Under normal physiological conditions, cells can regulate cell functions through free radicals produced.
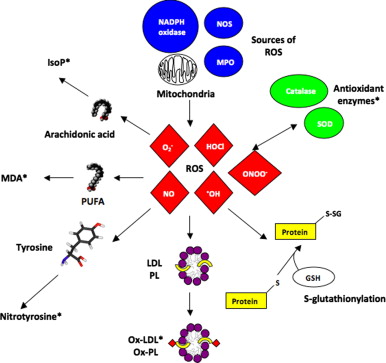
It is well known that exposure to ROS levels above the steady-state threshold has been identified as the main cause of damage to cellular macromolecules. Among them, the most sensitive biological component to ROS damage is DNA, especially mitochondrial DNA. As we all know, mitochondria are the main source of oxidants in cells. The damage of oxidative stress to DNA molecules is particularly evident in the break of the double-stranded helix and the change of the nitrogen base. The most studied DNA damage is the formation of 8-OH-G. Generally, for proliferating young cells, damage can be repaired more effectively by using base or nucleotide excision repair pathways and homologous recombination. However, in cells derived from elderly individuals, these repair pathways are less efficient, which is the first step leading to an increased incidence of carcinogenesis and mutagenesis.
In addition to DNA, proteins in the body are also affected by elevated ROS levels in terms of structure and function. Among them, the most easily oxidized components are the cysteine and methionine residues of the protein side chain. This is due to the formation of reducing disulfide bonds between the thiol groups of the protein. Normally, the proteasome in the body is responsible for breaking down oxidized proteins. However, as cells begin to age, the level of proteasome activity will be greatly reduced, leading to the accumulation of these oxidized proteins in the cell, which in turn leads to the activation of cell death pathways.
The last biological component to which cells are particularly sensitive to oxidants are lipids, especially the fatty acid residues of phospholipids in cells. The lipid peroxidation process involves various carbon-carbon bonds of free radical substances and produces products similar to hydroperoxides, which have been identified as potential indicators of oxidative stress in various tissues. Free radicals have the ability to damage cell membranes and cell integrity. From a macro point of view, the oxidation of lipids will change fluidity and cause severe physiological and membrane damage, which is also obvious in certain diseases. At the cellular level, ROS and OS induce a variety of related cell fates, such as apoptosis, necrosis, autophagy, and senescence, which may adversely affect tissue structure and function.
Since oxidative stress markers are necessary for accurate evaluation to study various pathological conditions and evaluate the effectiveness of drugs, biomarkers that can be used to evaluate oxidative stress have attracted much attention. From a clinical point of view, the use of biomarkers to assess the degree of oxidative stress is very valuable. The markers found in blood, urine and other biological fluids may provide information of diagnostic value, but it would be ideal if the organs and tissues suffering from oxidative stress can be directly observed in a way similar to scanning imaging. In recent years, attempts have been made to realize this idea using electron spin resonance technology, but it will take time to apply this method to humans. Since oxidative damage is bound to exist in the human body, some of its components may be damaged by free radicals, so oxidation products are usually used as markers. Many markers have been proposed, including lipid peroxides, malondialdehyde and 4-hydroxynonenal as markers of lipid oxidative damage. Isoprostaglandins are the products of arachidonic acid free radical oxidation; 8-oxoguanine (8-hydroxyguanine) and thymine diol can indicate DNA oxidative damage; and various oxidation products of protein and amino acid oxidation, Including carbonyl protein, hydroxybutyrin, hydrovaline and nitrotyrosine. Even in relatively early studies, lipid peroxides have been evaluated in clinical samples, and the analysis and detection methods for this substance have been improved. Among them, substances used to measure the reaction with thiobarbituric acid have been widely used in clinical and experimental research. Such substances have become the most commonly used indicators of oxidative stress, partly because lipid peroxidation is an important mechanism for cell membrane destruction. Lipid peroxidation is a chain reaction through which unsaturated fatty acids in cell membranes are oxidized. When hydrogen atoms are removed from fatty acid molecules for some reason, free radical chain reactions will proceed. Therefore, free radicals that can participate in the extraction of hydrogen atoms from lipids include hydroxyl (HO), hydroperoxide radicals (HOO), lipid peroxy radicals (LOO) and alkoxy radicals (LO). Metal-oxygen complexes, especially iron-oxygen complexes, are also important in the body. Once started, the peroxide chain reaction will propagate itself. The process of generating lipid radicals (L) from lipids (LH) is called chain initiation reaction. The lipid radicals (L) thus produced immediately react with oxygen to form LOO, which attacks another lipid and removes hydrogen atoms from it, resulting in the formation of lipid hydroperoxide (lipid peroxide; LOOH) and another An L. L also reacts with oxygen and forms LOO. LOO attacks another lipid to generate lipid peroxide, so as the chain reaction progresses, lipid peroxide will accumulate.
Among substances that protect human lipids from peroxidation, vitamin E is considered the most important. This vitamin has attracted widespread attention as an antioxidant because it can scavenge lipid peroxidation free radicals, thereby preventing the propagation of free radical chain reactions. The lipid peroxy radical removes a hydrogen atom from the phenyl group of vitamin E and has stabilized the hydrogen atom. In turn, vitamin E is converted into free radicals, which are stable and less reactive. Therefore, such free radicals derived from vitamins are unlikely to attack lipids and perpetuate the chain reaction. Instead, it can react with another peroxy radical and become stable as a result. This antioxidant reaction protects biological membranes from free radicals and lipid peroxides. However, despite the presence of a sufficient concentration of vitamin E, lipid peroxides are still produced in the plasma.
Therefore, plasma vitamin E level seems unlikely to be a useful biomarker of oxidative stress. In addition, vitamin E is fat-soluble, so its blood level varies according to the lipid content. Studies have found that when encountering oxidative stress, vitamin C decreases first, followed by coenzyme Q-10 (Panthenol 10). This indicates that vitamin C and panthenol 10 are the most sensitive antioxidants to oxidative stress. Vitamin E is an important antioxidant, so it can be protected by vitamin C and panthenol 10.